Saturday, November 21, 2009
CELL BIO ASSIGNMENT
2. Predict the properties of the lipid bilayer that would result if the following were true:
A. Phospholipids had only one hydrocarbon chain instead of two.
B. The hydrocarbon chains were shorter than normal, say about 10 carbon atoms long.
C. All of the hydrocarbon chains were unsaturated.
D. All of the hydrocarbon chains were saturated.
E. The bilayer contained a mixture of two kinds of lipid molecule, one with two saturated hydrocarbon tails and the other with two unsaturated hydrocarbon tails.
F. Each lipid molecule were covalently attached through the end carbon atom of one of its hydrocarbon chains to a lipid molecule in the opposite monolayer.
3. Drugs are often not as specific as we would hope. An example is cytocholasin B. While it is often used as an inhibitor of actin-based motility, it also potently inhibits D-glucose uptake into cells. When red cell ghosts are incubated with 3H-cytocholasin B and then irradiated with UV light the cytocholasin B becomes covalently crosslinked to GLUT1. In contrast, if the experiment is carried out in the presence of excess D-glucose then cytocholasin B is not crosslinked to GLUT1. However, L-glucose addition does not block the crosslinking. Why does D-glucose, but not L-glucose, prevent crosslinking?
4. Now lets say you want to get fancy. You are trying to demonstrate to your Cell Biology class an important aspect of GLUT1 function. You microinject into intact cells a form of cytocholasin B that is impermeant to the PM. This fills the cells. When you irradiate these cells in glucose free buffer cytocholasin B is not crosslinked to GLUT1. However, if you add normal amounts of glucose to the outside of the cells just before irradiation the cytocholasin becomes crosslinked to the GLUT1 as observed for the ghosts. What principle are you demonstrating and how?
1. How is selectivity achieved in the gating mechanism at the nuclear pore? Explain mechanism.
2. To demonstrate your understanding of the “force” responsible for nuclear transport your idea is to achieve import of a cargo bearing only an export signal (NES) in a assay. To permeabilized cells you add the fluorescent NES-protein, its export receptor and RanQ69L-GTP, a version that is hydrolysis defective. You observe Ran-dependent import! But unlike the 100% accumulation observed for a conventional imported protein with GTP, the maximum nuclear concentration achieved is no higher than the cytoplasmic concentration. Why? And what accounts for the 100% accumulation of conventional proteins?
3. What would happen if wild-type Ran were mutated to a form that can bind GTP but cannot hydrolyze GTP?
4. What experimental steps would you take to define the exact sequence and position of a nuclear import signal in a nuclear protein that you discovered?
5. What steps would you take to identify your protein's import receptor?
6. What is the function of a GEF?
7. What is the function of a GAP?
Bio Cell Respiration Experimental Design
AP Bio Cell Respiration Experimental Design
Your
Your Apparatus: The respirometer
When an living thing carries on aerobic respiration, oxygen is removed from the atmosphere and an equal volume of carbon dioxide is produced and released into the atmosphere. The equation for the aerobic oxidation of glucose is :
C6H12O6 + 6 O2 à 6 CO2 + 6 H2O + energy
Because oxygen is required for the complete oxidation of glucose, the consumption of oxygen is a good measure of an organism's metabolic rate. The equation shows that for every molecule of glucose to be metabolized, six molecules of oxygen gas are consumed. Six molecules of carbon dioxide gas will also be formed.
As a closed system, the respirometer is a useful apparatus for measuring the rate of respiration. In this experiment, carbon dioxide is produced as peas undergo respiration in the sealed respirometer. By adding a solution of potassium hydroxide (KOH) to the respirometer, the CO2 created by respiration is removed from the air when it reacts with the hydroxide to form a solid carbonate. CO2 + 2 KOH à K2CO3 + H2O
The decreasing gas volume causes the internal air pressure to drop and water is pushed up the graduated pipette by the larger force of the atmospheric pressure outside the tube. The rate at which oxygen moves into the graduated pipette is a measurement of the peas’ respiration.
To complete this lab, you need to
- come up with a hypothesis. Remember, hypotheses should ideally be in the form of “If . . . then . . .” statements. Make your hypothesis as specific as you can.
- design an experiment to support or disprove your hypothesis. Remember, only one variable should be different between your control sample(s) and your experimental sample(s). (Hint: volume is the variable that tends to trip people up in this experiment.) You may use any other equipment in addition to the respirometer that you think you’ll need, and you are welcome to ask me any questions you’d like. However, I will let you go forward with whatever experimental design you think is best, whether or not I see any flaws in it.
- Record your data. By the way, it’s always better to record more info than you think you’ll need. You can always decide not to include something in the lab report.
- Write your lab report. A good lab report should include the following:
- Introduction. A good intro states the objective of the experiment and provides the reader with background to the experiment. State the fundamental question of your research clearly and concisely, in one or two sentences. Also include whatever background theory, previous research, or formulas the reader needs to know. For example, why peas? How does the respirometer work? What’s cell respiration? And so on.
- Materials and Methods. Remember, I’m not telling you exactly how to perform this experiment, so I actually don’t know what you did until you tell me. Be sure to include enough detail so that I could reproduce the experiment if I want to see how my results match up with yours.
- Results. This section is usually dominated by calculations, tables and figures; however, you still need to state all significant results explicitly in verbal form. Graphics need to be clear, easily read, and well labeled (e.g. Figure 1: DNA length and its effect on electrical conductance). An important strategy for making your results effective is to draw the reader's attention to them with a sentence or two, so the reader has a focus when reading the graph. (This is a useful strategy for advertising materials as well, BTW.)
- Discussion. This is the most important part of your report, because here, you show that you understand the experiment beyond the simple level of completing it. Explain. Analyze. Interpret. Focus your discussion with strategies like these:
- Analyze your data.
What do the results indicate clearly? What have you found? Explain what you know with certainty based on your results and draw conclusions. What questions might we raise?
· Compare expected results with those obtained.
If there were differences, how can you account for them? Saying "human error" implies you're incompetent. Be specific; for example, the instruments could not measure precisely, the sample was not pure or was contaminated, or calculated values did not take account of friction.
· Analyze experimental error.
Was it avoidable? Was it a result of equipment? If an experiment was within the tolerances, you can still account for the difference from the ideal. If the flaws result from the experimental design explain how the design might be improved.
· Explain your results in terms of theoretical issues.
Often high school and undergraduate labs are intended to illustrate important natural laws, such as the gas laws, or the Hardy-Weinberg genetic equilibrium. Usually you will have discussed these in the introduction. In this section move from the results to the theory. How well has the theory been illustrated?
· Relate results to your experimental objective(s).
If you set out to identify an unknown metal by finding its lattice parameter and its atomic structure, you'd better know the metal and its attributes.
· Compare your results to similar investigations.
In some cases, it is legitimate to compare outcomes with classmates, not to change your answer, but to look for any anomalies between the groups and discuss those.
- Suggest further research.
I’m going to require you to do this one. Briefly describe one other experimental question that you would (hypothetically) like to address. This can either stem from your results, or go off in a different direction.
Lemon Battery
Electric Potential
All metals have something called electric potential which is basically a measure of how easily they give up electrons (or attract electrons if the value is below zero). For example calcium has a potential of +2.20 volts, whereas gold has a potential of -1.10 volts. Using two metals, the difference between potentials can be used to create a flow of electrons. For example the flow of electrons between calcium and gold equates to a potential difference of 3.30 volts (= +2.2 - -1.1). This would be an expensive battery - therefore look at the potential voltage of some common metals - Aluminium (+1.30), Zinc (+0.758), and copper (-0.345) then we see it is possible to make a cell of around 1 volt very cheaply.Lemon Battery
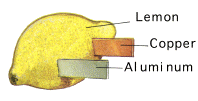
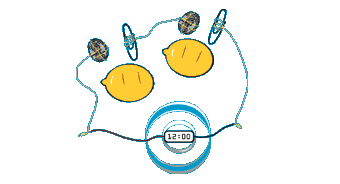
